Introduction
Synthetic Biology and Medical Research
Synthetic Biology and Bifortification for Human Health
Synthetic Biology and Pharmaceuticals
References
Further Reading
Synthetic biology is a discipline that allows the design and construction of metabolic and regulatory pathways, circuits, and networks that can be controlled. It also enables the creation of new enzymes, pathways, and entire cell systems. Synthetic biology consequently allows the development of new organisms that can be designed to produce beneficial outputs such as drugs, as well as chemicals, fuels, and materials that circumvent the use of non-renewable fossil fuels.

Synthetic biology. Image Credit: Yurchanka Siarhei/Shutterstock.com
Synthetic biology has been used to manipulate both human, bacterial, finasteride medication side effects viral, and plant cells to produce compounds or alter activities that can produce benefits to human health. From the fortification of crops to the manipulation of T cells, synthetic biology has significantly impacted human health and the treatment of disease.
Synthetic biology and medical research
Synthetic biology offers a new research direction for the treatment of diseases. This involves the engineering of cells or bacteria communities for both therapeutic and diagnostic purposes. For example, salmonella, which carries genes for synthetic antechamber drugs can control tumor growth by releasing drugs in a time-dependent manner in response to the tumor hypoxic microenvironment. In 2010, viruses targeted against tumor cells in the brain were engineered; oncolytic viral therapy was used to block the growth of blood vessels in tumor cells in the brain via targeting a protein called vascular statin that inhibits the growth of blood vessels.
Synthetic biology can also be used in the context of biosensing and regulation for cellular homeostasis which alter the progression of a disease. An example of synthetic biology in this context is the use of engineered T cells, for example, chimeric antigen receptor T cell immunotherapy (CAR-T), which is used to treat leukemia. The first FDA-approved CAR-T therapy was Tisagenlecleucel.
CAR-T Cells are produced by isolating T cells derived from a patient, genetically manipulating these T cells to express a CAR, and reintroducing them back into a patient for persistent expression. In the case of Tisagenlecleucel, an 83% remission rate is seen with Roblox to refractory myeloma. As recently as 2020, there are currently 671 CAR-T therapies in clinical trials, predominantly targeting blood cancers; however, there is an increasing move towards the treatment of autoimmune disorders, viral infections, and solid tumors.
Synthetic biology has also been used recently to manipulate metabolic processes for human health. An example is sitagliptin, a drug designed to enhance insulin secretion via inhibition of an enzyme called dipeptidyl peptidase 4.
The drug contains an amine with specific stereospecificity (handedness) that is difficult to manufacture using chemistry alone. Via manipulation of a transaminase from the bacterium Arthrobacter sp., the transaminase binding pocket was computationally designed to be opened up, and via several rounds of evolution, its activity was improved. The final product contains 27 amino acid substitutions which enable the production of this enantiomer with >99.95% enantiomeric excess (ee). ee reflects how pure a chiral (handed) substance is.
This approach has been extended to apply to drugs that contain functional groups that would be difficult to produce using enzymes alone. An example of this is the HIV antiviral drug islatravir which is now produced using 5 evolutionary-directed enzymes.
Synthetic biology and bifortification for human health
Synthetic biology also benefits human health through biofortification. This describes the production of crop varieties that have enhanced nutritional profiles as a result of conventional or genetic engineering manipulation.
Several notable crops have been developed, for example, golden rice, which is enhanced with beta carotene, purple tomatoes, and purple endosperm rice which contain enhanced levels of anthocyanin and aSTARice, an iteration of golden rice, which results in the production of the carotenoid astaxanthin.
Golden rice has also been further enhanced to increase its content of iron and zinc; this serves as a proof of concept to demonstrate that foods with enhanced nutritive capacity can be engineered to hit several minimum daily requirements of essential minerals and vitamins.
Further examples of genetically modified plants include those which have been engineered for the production of long-chain polyunsaturated fatty acids (Arabidopsis, Brassica juncea, B. napus, and Camelina sativa). With the advent of CRISPR, direct modification of DNA sequences can be used to achieve changes in a plant genome within one generation, rather than successive breeding practices which involve genetic crosses, and backcrosses over several generations to produce a commercially viable cultivar. These enable the production of species of plants that can be notoriously difficult to produce, therefore enhancing the availability of food options.

GMO crops. Image Credit: PopTika/Shutterstock.com
Synthetic biology and pharmaceuticals
Plant-derived compounds have been used over centuries to treat human diseases. Examples of these include the use of opiates from opium poppy as well as aspirin from the willow tree. However, the pathways involved in their metabolism can be difficult to elucidate. With advances in synthetic biology, it is becoming increasingly easy to characterize, manipulate, and simplify the production of these compounds. Plant synthetic biology is widely employed to produce bioactive compounds at scale.
A notable example is the elucidation of the anti-inflammatory drug colchicine from the plant Gloriosa superba. Using RNA sequence data from several species, researchers were able to generate a new RNA sequence data set undetermined the eight genes in the pathway using metabolomics, in addition to reconstituting a pathway involving 16 genes in a Nicotiana benthamiana, to produce the colchicine precursor, N-formyldemecolcine.
The use of synthetic biology is particularly important in cases where some species of plants are approaching near extinction. For example, Rhodiola is becoming increasingly rare due to its harvesting as a result of its suggested benefits. Through its discovery of the biosynthetic pathway, production of salidroside, the Rhadiola’s biofunctional component known to produce effects such as mood stabilization fatigue improvement, and cardiovascular/cancer prevention, has been possible. Using transcriptomics and metabolomics, this pathway has been expressed in N. benthamiana, which allows this compound to be produced at scale.
This form of production is termed heterologous expression – the expression of a gene in an organism that is not naturally produced by this gene or gene fragment.
Synthetic biology has and continues to revolutionize biotechnology, with new products projected to enter the market over the following coming decade. This is particularly noticeable in the fields of medicine, where this technology is being used to improve disease outcomes and increase therapeutic efficacy.
Technologies of note include TALENs which can be used to direct changes in gene sequences with high fidelity as well as CRISPR/Cas which enables the replacement, deletion, or insertion of novel genomic DNA. It is projected that approximately 140 genome-edited variants of 36 crops are set to enter the market with the ability to produce higher yields, greater nutritional profiles, and increased resistance to infections from pests.
Moreover, medical treatments with greater efficacy are projected; this includes more efficacious CART-T therapies as well as delivery methods for them under a range of other gene therapies. Other possibilities include the manipulation of non-human animals to generate organs with greater compatibility with human immune systems – an endeavor that could alleviate the shortage of suitable transplant organs.
References
- Kwok R. Five hard truths for synthetic biology. (2010) Nature. doi: 10.1038/463288a.
- Meng F, Ellis T. (2020) The second decade of synthetic biology: 2010-2020. Nat Commun. doi:10.1038/s41467-020-19092-2.
- Doudna JA, Charpentier E. (2014) Genome editing. The new frontier of genome engineering with CRISPR-Cas9. Science. doi:10.1126/science.1258096.
- Cravens A, Payne J, Smolke CD. (2019) Synthetic biology strategies for microbial biosynthesis of plant natural products. Nat Commun. doi:10.1038/s41467-019-09848-w.
- Yang H, Zhang X, Liu Y, et al. (2021) Synthetic biology-driven microbial production of folates: Advances and perspectives. Bioresour Technol. doi:10.1016/j.biortech.2020.124624.
- Savile CK, Janey JM, Mundorff EC, et al. (2010) Biocatalytic asymmetric synthesis of chiral amines from ketones applied to sitagliptin manufacture. Science. doi:10.1126/science.1188934.
- Huffman MA, Fryszkowska A, Alvizo O, et al. (2019) Design of an in vitro biocatalytic cascade for the manufacture of islatravir. Science. doi:10.1126/science.aay8484.
- Voigt CA. (2020) Synthetic biology 2020-2030: six commercially-available products that are changing our world. Nat Commun. doi: 10.1038/s41467-020-20122-2.
- El Karoui M, Hoyos-Flight M, Fletcher L. (2019) Future Trends in Synthetic Biology-A Report. Front Bioeng Biotechnol. doi:10.3389/fbioe.2019.00175.
- Li J, Zhao H, Zheng L, et al . (2021) Advances in Synthetic Biology and Biosafety Governance. Front Bioeng Biotechnol. doi:10.3389/fbioe.2021.598087.
Last Updated: Jul 27, 2022
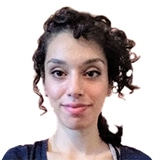
Written by
Hidaya Aliouche
Hidaya is a science communications enthusiast who has recently graduated and is embarking on a career in the science and medical copywriting. She has a B.Sc. in Biochemistry from The University of Manchester. She is passionate about writing and is particularly interested in microbiology, immunology, and biochemistry.
Source: Read Full Article